CARBON MANAGEMENT WILL PLAY A BIG ROLE IN MEETING CANADIAN CLIMATE TARGETS, BUT A NEW APPROACH IS NEEDED TO REDUCE PROJECT COSTS.
- International groups and the Canadian federal government agree that carbon management (i.e. capture, removal, storage and use of emitted CO2) will be a critical part of reaching net-zero emissions.
- Canada is currently a world leader in carbon management, with the third most active projects (after the U.S and China). Data shows Canada will have roughly 30 MtCO2e of installed capture capacity in place by 2030, even with the cancellation of the Genesee CCS project.
- To align with the low-end of projections for required capacity to reach net-zero, CCS capacity needs to grow at 5%–6% year-on-year from 2030–2050. Direct air capture would need to grow at roughly 30% year-on-year in the same timeframe.
- Despite having the storage potential, expected infrastructure and clean electricity supply necessary to support carbon management in coming years, the sector is not growing as fast as it needs to.
- A primary reason for low installation is high hurdle rates owing to factors like the high costs of technologies, uncertainty and limited revenues generation potential.
- Canada should prioritize improving project economics by rapidly deploying carbon management solutions to speed up learning curve effects and leverage economies of scale, and supporting greater use of captured/removed CO2 (alongside permanent storage).
- Strengthening emerging supply chains, continuing to develop shared storage and transport infrastructure, and forging deeper partnerships with the U.S. could all reduce long-run costs.
- Reducing the overall costs of carbon management solutions could serve as a core Canadian contribution to global climate action.
EMISSIONS NEED BETTER MANAGEMENT
Emissions growth may be slowing, but the world is not on track for net-zero by mid-century. This is clear in climate commitments, fossil fuel demand outlooks and warming forecasts. Despite expectations that global emissions will peak in mid-2020s, countries representing only 42% of global emissions have pledged net-zero by 2050. Only four of the top ten global emitters expect to reach net-zero by 2050, with the remainder pledging past that date or setting no net-zero target. Additionally, to remain on track with the International Energy Agency’s (IEA) Net-Zero by 2050 Scenario, global oil demand would need to decline by 26.6 – 78.4 million barrels per day by 2045 below levels projected in other IEA and OPEC scenarios. Natural gas demand would similarly need to decline (chart 1 and chart 2). Sustaining higher rates of emissions will have consequences for warming. The UN’s Annual Emissions Gap report identified current emissions trajectories have the world on track for 2.9°C above pre-industrial levels this century. Emissions would need to decline by 28% – 42% below expected 2030 levels for alignment with 1.5°C – 2°C pathways.
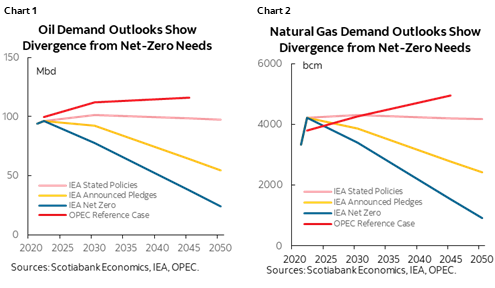
Carbon management will be a big part of tackling both stocks and flows of GHGs. Carbon management refers to either capturing CO2 from a point-source emitter, or removing CO2 from the atmosphere. Capturing CO2 from a facility can be achieved before or after combustion occurs at a specific facility (referred to as CCS/CCUS). Carbon dioxide removal is conducted through natural, engineered or hybrid means, with pathways ranging from mass tree planting/reforestation (natural), to changing ocean alkalinity to accelerate carbon sequestration rates (hybrid), to the development of direct air capture (DAC) facilities that extract CO2 from ambient air (engineered). Once CO2 is captured or removed, it can either be stored out of the atmosphere, or resold (i.e. “used”) as an industrial input. Potential storage mediums range from underground caverns to seawater to concrete. Use of CO2 is already common practice. 230Mt CO2 were used as industrial inputs globally last year, with over 90% dedicated to urea manufacturing (inputs for fertilizer) and enhanced oil recovery (oil production). The Intergovernmental Panel on Climate Change (a UN body) states that credible pathways for net-zero will need to include carbon management to both reduce the stock of greenhouse gases in the atmosphere, and mitigate ongoing flows from hard-to-abate sectors (i.e. aviation, shipping, industrial processes, etc.). IEA scenarios estimate 401 – 6040 MtCO2e could be captured globally by 2050, and 89 – 1710 MtCO2e could be removed (the Net-Zero by 2050 Scenario has carbon removals responsible for roughly 15% of total emissions reductions by 2050). In these estimates, over 95% of CO2 captured or removed is permanently stored.
CANADA’S PLANS FALL SHORT OF ITS NEEDS
Canadian scenarios note carbon management will be required at an enormous scale. While net-zero scenarios from a range of government and third-party groups diverge on the amount of carbon management that could be required in different futures to keep Canada on track, they all show substantial growth above today’s levels (chart 3). The Canada Energy Regulator’s 2023 Canadian and Global Net-Zero Scenarios have CCS responsible for 60–80 MtCO2e mitigated in Canada by 2050, and calls for DAC to remove 46–55 MtCO2e from the atmosphere by the same date (for context, Canadian GHGs have seen a net decline of 54MtCO2e since 2005). Environment and Climate Change Canada’s Long-Term Scenarios (a scenario modelling exercise submitted to the UN by the federal government) estimate that direct air capture could account for up to 201 MtCO2e of annual emissions reductions by 2050. Some third-party scenarios call for CCS and DAC to account for roughly 30%–40% of total GHG mitigation below current levels by 2050. Although exact contributions from natural, engineered, hybrid or captured pathways may shift as mitigation efforts advance, a range of estimates clearly indicate carbon management will play a significant role in reaching net-zero emissions.
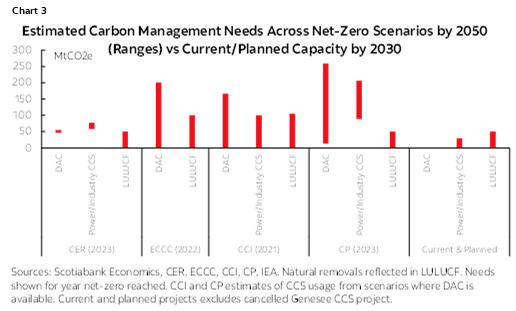
Canada is not starting from scratch. Canada currently has the third most operational carbon management projects globally (after US and China), but this amounts to eight capture, removal, transport and storage projects. There are plans for more—four projects are under construction, and an additional 62 are planned by 2030 for a total of 29.7MtCO2e of operational and planned capture capacity. Available data shows which infrastructure types and regions are currently attracting investment. Of projects whose capacity has been announced, Canada has roughly 5.3x more planned capacity for transport and storage than capture (158Mt to 30Mt). Data shows 28 carbon management hubs (locations that have shared transportation and/or storage infrastructure) are planned for over 70 named projects. 80% of current and planned projects are in Alberta, 11% are in Saskatchewan, and the rest are distributed in different provinces. Within each province, planned capture capacity by 2030 will be sufficient to sequester 2.7% of industrial GHGs in BC, 3.9% in Ontario, 8.4% in Saskatchewan and 19.5% in Alberta. Canada has only two DAC projects (251 Kt of removal capacity) planned by 2030 currently, although this figure will likely increase.
Canada has potential for far more, but will need to build out the infrastructure to realize it. Even when accounting for just one potential storage medium (saline aquifers), provinces where at least one carbon capture project is being built have the geological potential to sequester roughly 1600% – 800,000% of their current annual industrial emissions volumes. Accessing these storage sites requires transportation and/or proximity of large emitters to sites to improve feasibility and economics, which is not currently the case in all provinces (chart 4). Additionally, to ensure environmental benefits are not offset, engineered removal solutions will need to use emissions-free energy. Chart 5 illustrates mixed outlooks for each province’s blend of heavy industry emissions, large storage potential and emissions-free electricity generation capacity.
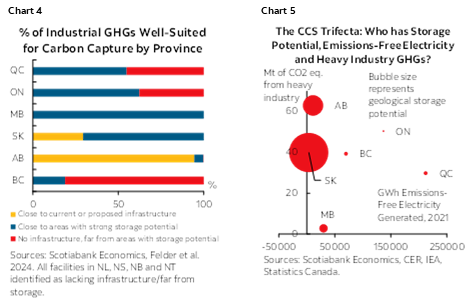
Even with planned projects and technical potential, carbon management is not growing as quickly as Canada needs to reach net-zero by 2050. To remain on track with meeting installation targets laid out in Net-Zero Scenarios by Canada’s Energy Regulator, capture capacity would need to grow by 4.7% – 6.2% annually from 2030 to 2050 (assuming all current planned projects are operational by 2030). This would entail installing 14–19 MtCO2e of capacity between 2030 and 2040, and 21 – 35 MtCO2e from 2040 to 2050. DAC capacity, beginning from a lower base, would need to grow by 29.8% – 30.9% annually in the same period (chart 6 and chart 7). DAC is currently set to experience no growth from 2026–2030, although this could change if additional projects are announced and operational by the end of the decade (if they are not, 45–54 Mt of DAC capacity will need to be installed in the 2030 to 2050 period to remain on track). As shown in chart 3, other reports call for more ambitious 2050 targets, and would require higher growth rates. Construction lead times for DAC facilities range from 2–6 years (on average) and the International CCS Knowledge Centre considers six years an accelerated timeframe for large-scale CCS projects, meaning project planning will need to begin swiftly to meet expected construction rates.
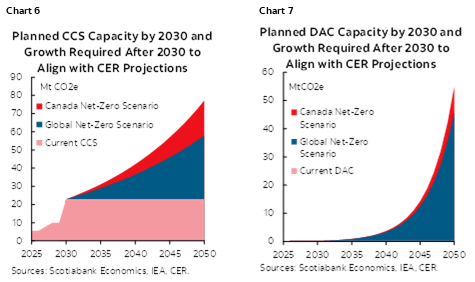
STICKER SHOCK IS THE TOPIC DU JOUR
A primary reason for slow growth thus far is high costs for capture solutions, which will have a material impact on the future outlook. A 2021 ask from Alberta’s provincial government for $30B from the federal government to deploy 30+Mt of capture capacity underscores the scale of costs identified when discussing the role of CCS/CCUS. These high costs are likely to impact adoption. Analysis from Canada’s Energy Regulator illustrates that higher CCS/CCUS costs would see slower uptake and declines in overall use within Canadian oil production by 2050. These estimates note lower deployments would have an impact on emissions (roughly 27%–33% higher by 2030 relative to lower CCUS cost scenarios, with a need to offset these emissions elsewhere) and in-situ oil production, which would be expected to be roughly 470,000 barrels per day lower (assuming emissions targets were adhered to). A 2024 report from Deloitte assessing the economic impacts of the proposed federal oil and gas emissions cap found that CCS’ high costs meant it was currently non-viable for higher-cost oil sands operators, but could remain attractive for lower-cost operators through 2040.
Engineered removals are also no free lunch. If CCS capacity is not developed and net-zero commitments still need to be met, non-captured emissions would need to be sequestered elsewhere. In scenarios with higher-cost CCS, Navius Research estimates 4%–12% higher DAC usage to offset reduced use of capture technologies. This would not eliminate cost challenges. The International Energy Agency notes DAC projects are currently the most expensive form of capture or engineered removal. While cost declines are expected, they will remain high. Developers of a recent major DAC project have expectations of cost declines to only $400–$600/tonne by 2030, and $200–$350/tonne by 2040.
High costs are present for a wide range of reasons, many of which can be tackled. It’s unclear exactly how much costs will decline for projects, and wide ranges of cost estimates make forward-looking analyses highly uncertain. Some have even gone so far to argue that cost declines for certain forms of carbon management will be minimal (or non-existent) given the complex and often bespoke nature of these projects. While we do not have a crystal ball, this seems unlikely. The challenges faced by carbon capture and engineered removals (high energy requirements and project costs, reliability concerns, etc.) are not insurmountable, and investment and policy to address the following should sufficiently address concerns and lead to cost declines:
- The development of best practices;
- Developing a skilled workforce;
- Building common carrier transportation and storage infrastructure;
- Reducing timelines for project approvals;
- Innovation around technologies to improve efficiency and minimize input costs;
- Scaling (and standardizing) both the manufacturing of components in carbon management supply chains, and the size of capture units themselves, and;
- Greater experience building carbon management technologies with similar applications (which has not been common over the technology’s history).
Project developers have echoed this. Developers of CCS plants on coal-fired generation stations have indicated capital costs could be reduced by at least 20% for future projects given what they learned building initial facilities, and CCS operators in Alberta have indicated future economies of scale for CO2 storage infrastructure would reduce operating costs. As noted above, cost declines are also expected for DAC. Achieving cost reductions would materially improve project economics through both reducing capital and operational costs, and lowering the opportunity costs associated with investments.
Discussions about carbon management’s economics also need to consider the other side of the ledger: revenues. For many projects, a key reason contributing to high cost-per-tonne figures is a lack of positive cash flow generated from value-added activities. Revenues from carbon management solutions can be generated through sales of captured carbon for industrial use, bilateral credit agreements, and sales of credits into environmental markets (voluntary or mandatory). These options are available for carbon removals or CCU/CCUS, but capture projects with only permanent storage have fewer options. If operating in a market with market-based compliance mechanisms (i.e. generation of credits that can be bought or sold for compliance purposes), then positive cash flow from the additional expense can take the form of credits for those in compliance, or incentives. In practice, this creates projects whose only revenue streams come from public resources or avoided compliance costs (meaning they are not revenues generated through value-added activities). These projects require either sufficiently elevated carbon costs and/or incentives to improve their economic feasibility (particularly if oil prices remain low long-term), limiting the options for generating cash flow.
These high costs (both realized and opportunity) and limited flexibility are influencing the policy debate. The combination of factors detailed above (and recent cancellation announcements) has led to calls for financial supports to advance projects. The presence of both federal tax credits (covering up to 37.5% – 60% of capital expenditures) and provincial grants (Alberta offers grants covering up to 12% of eligible capital costs) are not seen as sufficient by some project proponents. More support has been called for, including reducing future uncertainty that could adversely impact revenue streams (through measures like contracts for difference) and further cost supports. This perspective has been challenged by others, prompting a national discourse dominated by discussions of “who should pay” for capture solutions. This discourse may expand to include carbon removal solutions in future.
INVESTING IN THE FUTURE WE WILL NEED
Focusing on today’s costs makes sense, but the reality remains that carbon management is needed. Carbon management solutions, particularly capture and engineered removals, are expensive, can face technological uncertainty—and are increasingly essential to meeting climate goals. Their marginal costs may be high relative to alternative approaches to reducing emissions (particularly today), but lowering those costs will not happen automatically over time. If the costs of capturing, removing, transporting and storing CO2 are to decline moving forward, investment into the factors detailed above will be required. Some of these investments can be supported by private organizations, but many will also require public support. This will likely mean higher near-term marginal costs for GHG reduction, but could drive down total abatement costs associated with net-zero goals while simultaneously generating positive spillovers. In other words, if Canada’s action help lower technology/project costs, this could benefit both future Canadians (who may need even greater volumes of carbon management than expected if warming accelerates and/or other solutions are not adopted) and other countries with emission reduction targets. This may be of particular benefit to lower-income countries who have fewer public resources to dedicate to domestic decarbonization. Supporting cost declines for carbon management could even be a pillar of Canada’s contribution to global climate action.
A new approach is needed that advances the ultimate objective: Improving the economics for carbon management projects (and decarbonization by extension). A two-track strategy that considers both sides of the ledger should target the measures outlined above to achieve economies of scale and accelerate learning curves, and also address the revenue dimension. Greater investment should go towards CO2 utilization projects that offer additional revenue streams for capture and engineered removals projects. New uses in building materials, synthetic fuels (including jet fuel) and chemicals could offer growth markets and support additional investment. Final market size for these products will depend on a wide range of factors, but additional non-public revenue streams would likely improve the economics of both capture and engineered removals projects. This, in turn, could support greater deployment of carbon management solutions, accelerate cost declines, and subsequently drive down per unit costs as scaling occurs—even for future projects aiming solely to permanently store CO2.
If Canada installs more “U”, it will need to strategically build even more capture and engineered removals capacity than models currently call for to avoid offsetting climate benefits. Government and third party typically call for the vast majority of captured and removed CO2 to be permanently stored. However, if some Canadian capture and removals capacity that was once designated to store carbon is repurposed instead for utilization of carbon, this will mean less permanent storage occurs than previously expected. This could create challenges in net-zero accounting. The simplest solution to avoid this is to simply build even more capture and engineered removals capacity than models say is needed, with a recognition not all captured or removed carbon will be permanently stored. This will mean the sector needs to grow more rapidly than previously determined.
Canada can try to reduce costs by itself, but effects from learning curves and economies of scale will be more significant if it partners with other countries (i.e. the bigger, the better). In particular, deeper partnerships with the U.S. could prove beneficial, given their larger market and global leadership role in advancing carbon management projects. Discussions can build on existing efforts, but should emphasize specificity when priority setting. Comparing U.S. and Canadian planned projects shows only a small number of opportunities for sector-level best practice and knowledge sharing in the near-term, but individual project developers could still benefit (chart 8 and 9). The economics of a cross-border CO2 storage and transportation pipeline network should also be explored. In 2021, heavy industry and electricity providers that could adopt CCS/CCUS technologies in U.S. border states emitted roughly 596 MtCO2e. Connecting Canada’s growing carbon management sector with the estimated 11.4Mt of capture capacity and 42.1 Mt of transportation and storage capacity expected in U.S. border states by 2030 could further shared aims, and support technology adoption on both sides of the border. This would require harmonizing handling and regulatory standards, as well as solving interfacing challenges (i.e. managing ownership transfers and risk, etc.), but can build on historic collaborations such as the existing 330km CO2 pipeline operating between North Dakota and Saskatchewan.
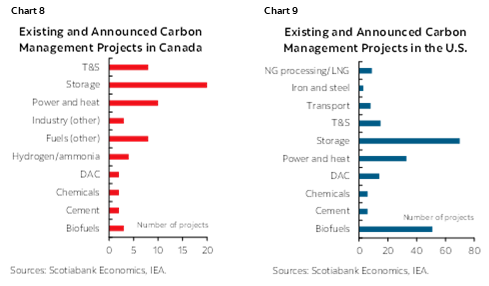
Long-term, Canada has the land, clean energy and storage potential to serve as an attractive hub for carbon management globally. If these attributes can be paired with expertise, international partnerships, credit market reforms (such as transparently publishing credit prices) and infrastructure, the sector could serve as an opportunity for investment and provide a global benefit for climate action. If Canada earns an early manufacturing edge, it may even prove a future source of export revenues, IP and foreign direct investment (i.e. other countries investing in carbon removal facilities built in Canada that leverage the country’s geological storage potential, infrastructure, skilled workforce and clean electricity). Capturing those benefits will take leadership, capital and strong management of carbon dioxide.
ANNEX
Methodology for CAGR modelling: 2024–2030 picture was determined using data from the IEA CCUS Project Database, concentrating on capture, CCS and full-chain projects to evaluate planned capture capacity. Date of project operations were taken as reported within the dataset, with the assumption operational dates are achieved. 2050 levels were estimated using the values identified for DAC and CCS for fossil and industrial usage from the Canada Energy Regulator’s Global and Canadian Net-Zero Scenarios. The compound annual growth rate required for current and planned installments by 2030 to reach 2050 levels for DAC and CCS/CCUS across both CER scenarios was calculated, then applied to assess the scale of installments required year-on-year from both technologies (measured in MtCO2e). Data reflects available capacity estimates for capture and DAC. Current or planned operations are assumed to remain operational until at least 2050. Figures do not account for storage, transportation or non-permanent CCU installments. Real capacity requirements may be higher, depending on whether existing facilities sell captured/removed CO2 for utilization purposes that have non-permanent storage implications, and/or operational challenges limit the use of facilities for any period of time (in this case, additional CDR capacity may be required on a compensatory basis, which was not accounted for in this analysis).
DISCLAIMER
This report has been prepared by Scotiabank Economics as a resource for the clients of Scotiabank. Opinions, estimates and projections contained herein are our own as of the date hereof and are subject to change without notice. The information and opinions contained herein have been compiled or arrived at from sources believed reliable but no representation or warranty, express or implied, is made as to their accuracy or completeness. Neither Scotiabank nor any of its officers, directors, partners, employees or affiliates accepts any liability whatsoever for any direct or consequential loss arising from any use of this report or its contents.
These reports are provided to you for informational purposes only. This report is not, and is not constructed as, an offer to sell or solicitation of any offer to buy any financial instrument, nor shall this report be construed as an opinion as to whether you should enter into any swap or trading strategy involving a swap or any other transaction. The information contained in this report is not intended to be, and does not constitute, a recommendation of a swap or trading strategy involving a swap within the meaning of U.S. Commodity Futures Trading Commission Regulation 23.434 and Appendix A thereto. This material is not intended to be individually tailored to your needs or characteristics and should not be viewed as a “call to action” or suggestion that you enter into a swap or trading strategy involving a swap or any other transaction. Scotiabank may engage in transactions in a manner inconsistent with the views discussed this report and may have positions, or be in the process of acquiring or disposing of positions, referred to in this report.
Scotiabank, its affiliates and any of their respective officers, directors and employees may from time to time take positions in currencies, act as managers, co-managers or underwriters of a public offering or act as principals or agents, deal in, own or act as market makers or advisors, brokers or commercial and/or investment bankers in relation to securities or related derivatives. As a result of these actions, Scotiabank may receive remuneration. All Scotiabank products and services are subject to the terms of applicable agreements and local regulations. Officers, directors and employees of Scotiabank and its affiliates may serve as directors of corporations.
Any securities discussed in this report may not be suitable for all investors. Scotiabank recommends that investors independently evaluate any issuer and security discussed in this report, and consult with any advisors they deem necessary prior to making any investment.
This report and all information, opinions and conclusions contained in it are protected by copyright. This information may not be reproduced without the prior express written consent of Scotiabank.
™ Trademark of The Bank of Nova Scotia. Used under license, where applicable.
Scotiabank, together with “Global Banking and Markets”, is a marketing name for the global corporate and investment banking and capital markets businesses of The Bank of Nova Scotia and certain of its affiliates in the countries where they operate, including; Scotiabank Europe plc; Scotiabank (Ireland) Designated Activity Company; Scotiabank Inverlat S.A., Institución de Banca Múltiple, Grupo Financiero Scotiabank Inverlat, Scotia Inverlat Casa de Bolsa, S.A. de C.V., Grupo Financiero Scotiabank Inverlat, Scotia Inverlat Derivados S.A. de C.V. – all members of the Scotiabank group and authorized users of the Scotiabank mark. The Bank of Nova Scotia is incorporated in Canada with limited liability and is authorised and regulated by the Office of the Superintendent of Financial Institutions Canada. The Bank of Nova Scotia is authorized by the UK Prudential Regulation Authority and is subject to regulation by the UK Financial Conduct Authority and limited regulation by the UK Prudential Regulation Authority. Details about the extent of The Bank of Nova Scotia's regulation by the UK Prudential Regulation Authority are available from us on request. Scotiabank Europe plc is authorized by the UK Prudential Regulation Authority and regulated by the UK Financial Conduct Authority and the UK Prudential Regulation Authority.
Scotiabank Inverlat, S.A., Scotia Inverlat Casa de Bolsa, S.A. de C.V, Grupo Financiero Scotiabank Inverlat, and Scotia Inverlat Derivados, S.A. de C.V., are each authorized and regulated by the Mexican financial authorities.
Not all products and services are offered in all jurisdictions. Services described are available in jurisdictions where permitted by law.